Wind has always been a source of power. It has propelled sail boats for at least 5,000 years, and turned windmills for perhaps 1,500 years. Even the concept of wind-generated electricity dates back to the first successful attempts in the late 1800s. But aside from the first few kilowatt-generating facilities in the 1970s, it's only since the last decade or so when wind power at scale finally took off: Total wind capacity rose fourfold from 159GW at the start of 2010 to 651GW at the end of 2019, and it is set to achieve 2,110GW by 2030 according to the Global Wind Energy Council. Wind is one of the fastest growing energy markets out there.
The terms "wind energy" and "wind power" refer to the energy extraction from the layers of the atmosphere that are closer to the earth's surface, where wind's movement (a.k.a. kinetic energy) is used to generate mechanical power and electricity. In a nontechnical, nonscientific way, you can think of it as a fan which instead of using electricity to make wind, it uses wind to generate electricity.
The unit economics relies on the workings of a single wind turbine, which are broadly classified as:
- Horizontal-Axis Wind Turbines: These are what most people picture when thinking of wind turbines. The vast majority have three blades and operate "upwind," with the turbine pivoting at the top of the tower so the blades face into the wind.
- Vertical-Axis Wind Turbines: These come in several forms and shapes. These turbines are omnidirectional, meaning they don’t need to be adjusted to point into the wind to operate.
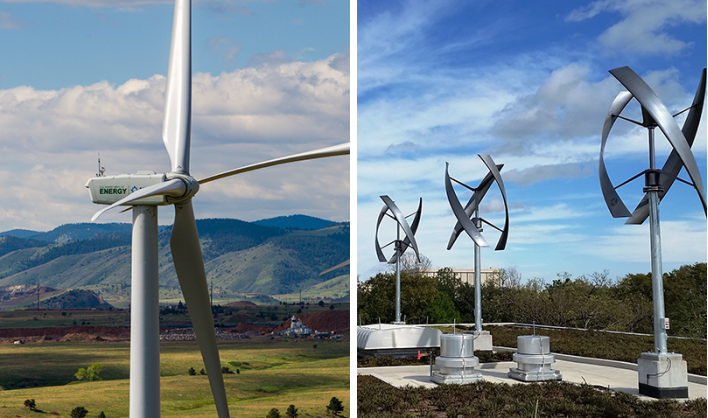
Most of the commercial wind farms use horizontal-axis turbines. Each turbine has a hub (tower) that lifts propeller-like blades up in the air. A blade is shaped so that when air blows, it splits the flow and creates two pressure profiles at the same time: lift and drag. The force of the lift is stronger than the drag and this causes the rotor to spin. The rotor connects to the generator, either directly (if it’s a direct drive turbine) or through a shaft and a series of gears (a gearbox) that speed up the rotation and allow for a physically smaller generator that feed AC power to the grid. For the sake of clarity, this video does a good job explaining the basics.
The math on wind turbines is pretty simple: Bigger is better (see next figure). This can be achieved in two ways. The first is with bigger rotors and blades to cover a wider area, increasing the capacity of the turbine, i.e., its total potential production. Rotor diameter is a measurement of the full sweep of the turbine’s blades (the diameter of the circle they define). All else being equal, greater rotor diameter means a turbine can harvest more wind.
The second way to produce more power is to get the blades up higher into the atmosphere, where the wind blows more steadily; that increases the turbine’s “capacity factor,” i.e., the amount of power it actually produces relative to its total potential (or more colloquially: how often it runs).
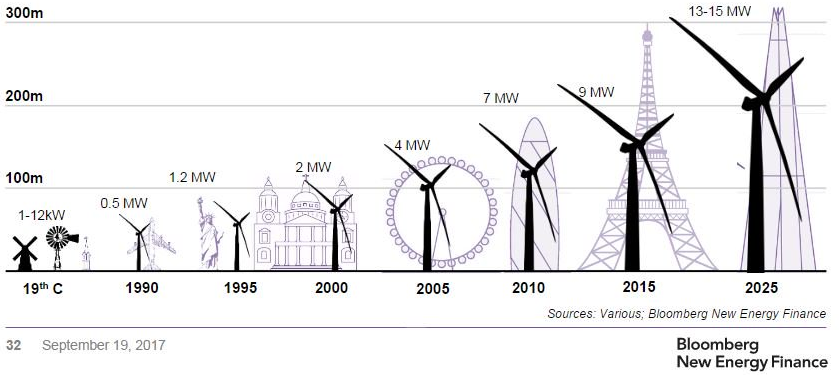
Bigger turbines harvest more energy, more steadily; the bigger they get, the less variable and more reliable they are, and the easier they are to integrate into the grid.
But on land, bigger isn't the real limitation. There are more pressing issues: transportation and infrastructure chokepoints, land-use concerns, worries about views, large birds, shadows, etc. Even the total height can be restricted by aviation concerns, which is why the median land-based turbine is less than 500 ft tall (150 m). This means the hub height has remained constant, although more power has been harvested by increasing the rotor diameter (see Figure below for the US market).
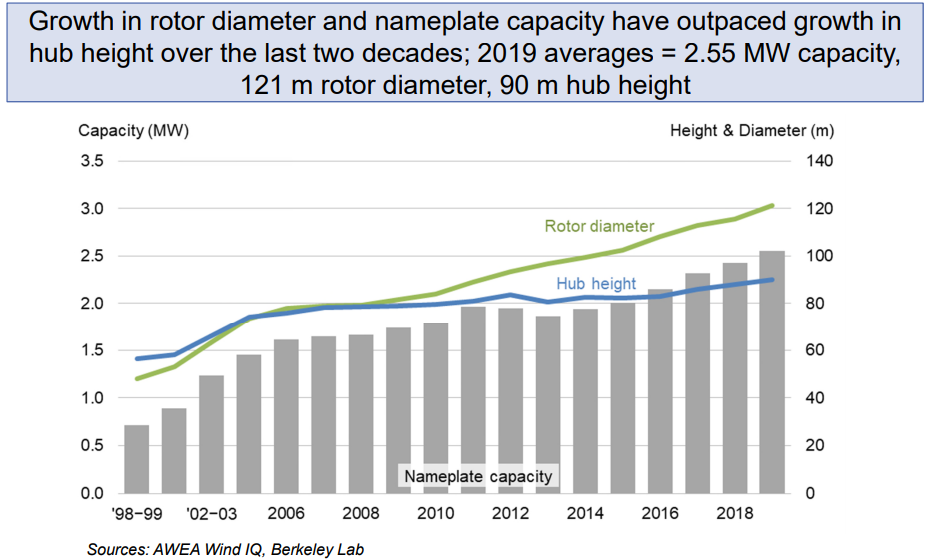
Offshore it's a different game and it's really what's fueling the growth in this market. With land barely in sight, the only limitation of wind turbines out in the sea is size and engineering, though some items still need to be transported.
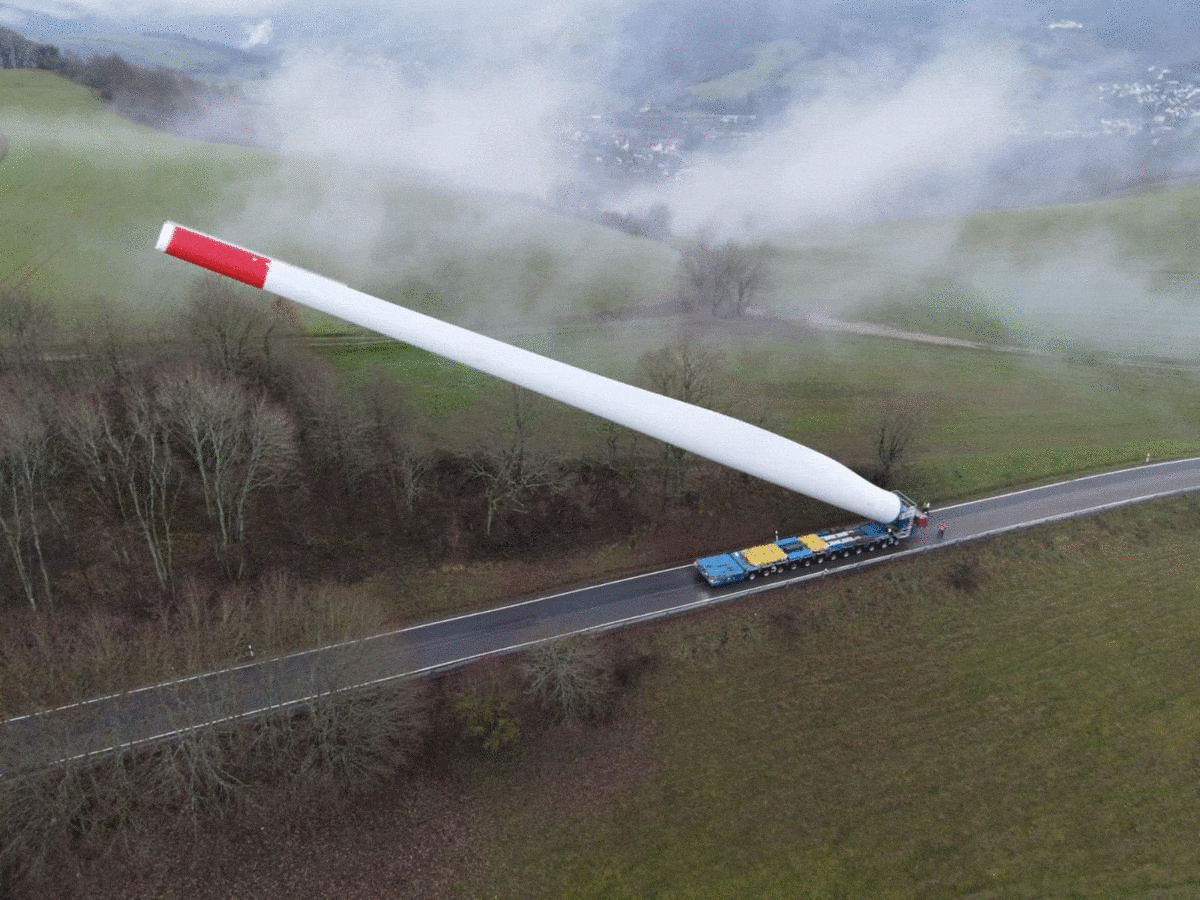
Performance Efficiency
A subject often misunderstood and overlooked regarding electricity generation are the concepts of electric generation capacity and capacity factor. First, let’s start with what the term “capacity” means when referring to electricity generation.
Capacity is the maximum electric output a generator can produce under specific conditions. Each power plant or generating facility has a “nameplate capacity” which indicates the maximum output that generator can produce. For example, if XYZ Power Plant has a nameplate capacity of 500 megawatts, it means the plant is capable of producing 500 megawatts operating at continuous full power.
The capacity factor is the ratio between what a generation unit is capable of generating at maximum output versus the unit’s actual generation output over a period of time. These two variables can be significantly different. Many generators do not operate at their full capacity all the time. A generator’s output may vary based on maintenance issues, weather conditions such as wind availability and/or as instructed by the electric power grid operator.
Wind turbines work by slowing down passing wind in order to extract energy. Hence they aren't fully efficient by design. In fact, the wind-electricity conversion is dictated by something known as the Betz limit, which states that at most 59.3% of the kinetic energy from wind can be used to spin the turbine and generate electricity. In reality, turbines cannot reach the Betz limit. Wind doesn't blow all the time, so a turbine doesn't run continuously all year-long. Thus, the typical capacity coefficient of a wind farm is approximately 30% for projects that were commissioned before 2011. Newer projects borderline 40-42%.
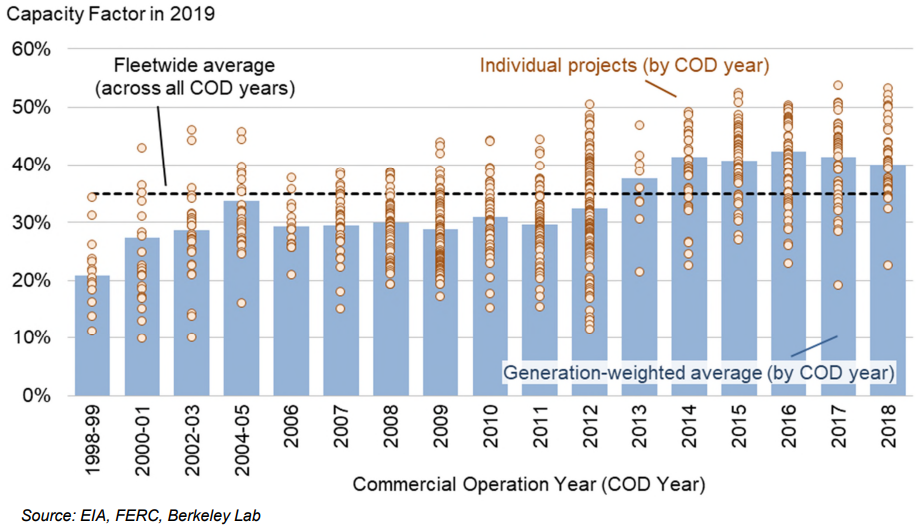
This apparent improvement in capacity factor among more recently built projects is impacted by several factors, including project location and the quality of the wind resource at each site, turbine scaling and design, and performance degradation over time.
On Risks
Tall, skinny, heavy things, placed in higher winds, tend to bend and flex. When long turbine blades bend, they can crash into the tower, or hub, like this Danish system did in 2008 after its “brake” failed and it spun out of control:
Or even the hub tower can break because of high wind, earthquakes, inadequate material selection, or poor installation practices, as you can see in the following pictures.
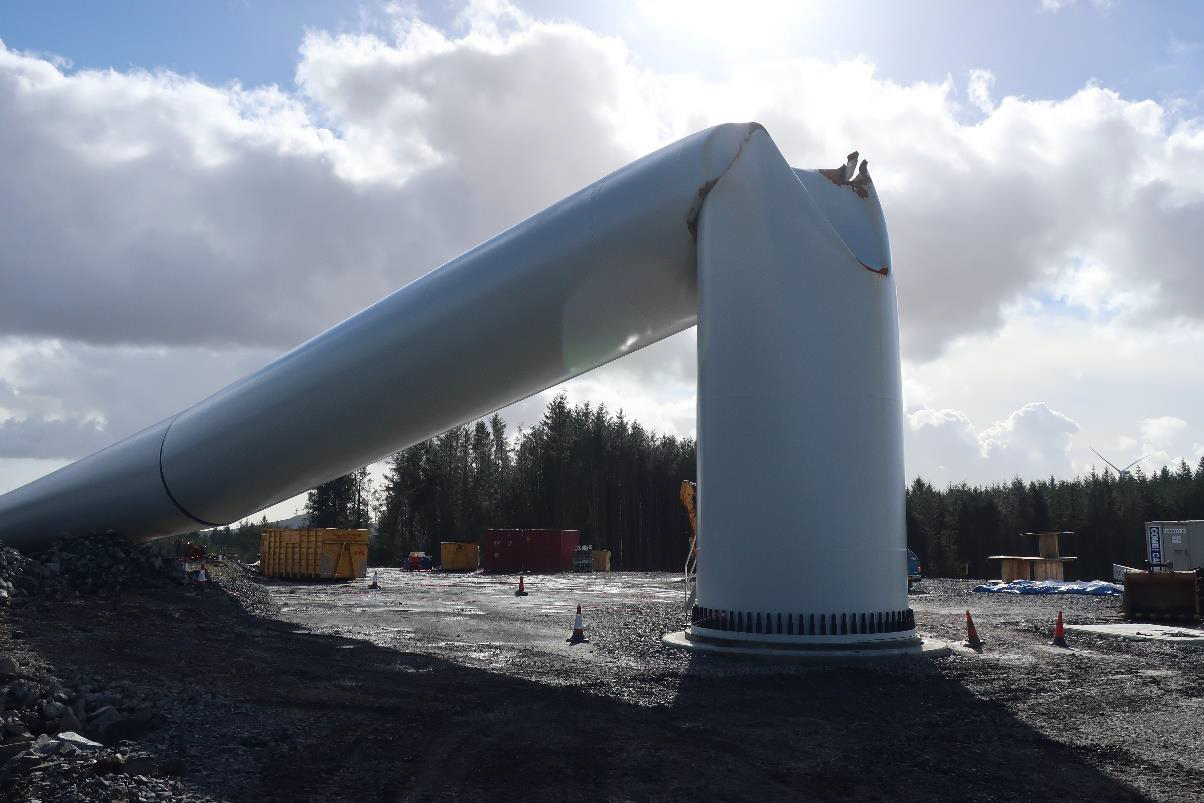
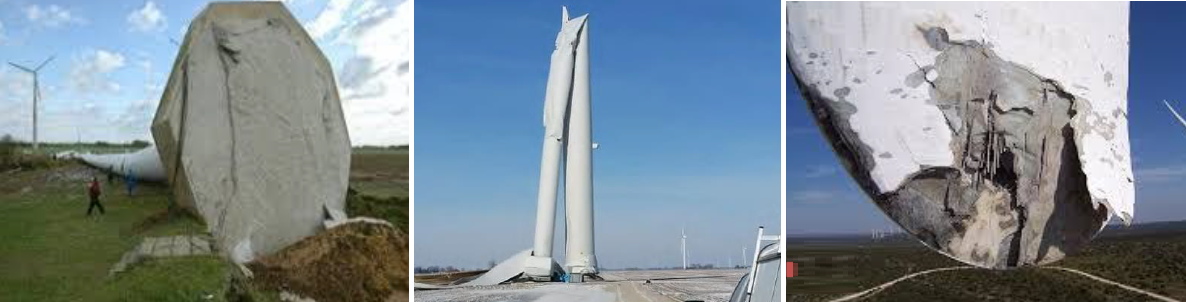
On the other hand, a turbine also has several mechanical and electrical components, as shown in the following picture. The risks increases with a growing growing fleet of older turbines that need to be managed and maintained. Notwithstanding, the number of technicians needed to do that work has not grown at the same pace.
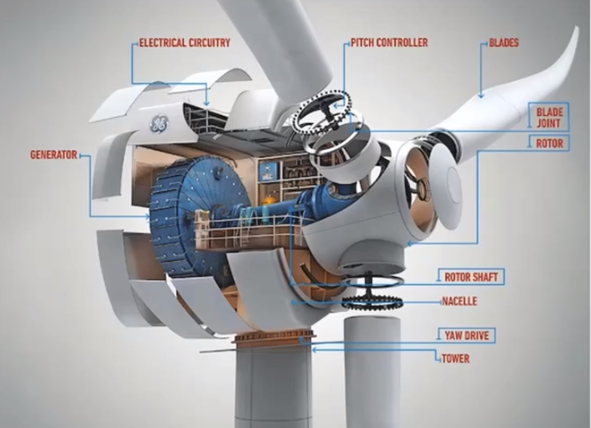
Experience will also tell us that anything using high-speed rotating equipment and electrical conversion can easily catch fire. In case you forget, you can watch the next video as a reminder.
The newer land-based turbine installation cost is between $1.5M and $3M, but replacing one after a catastrophic fire is likely to cost three or four times that. That could put it in the region of $8M to $9M. Offshore numbers can be starker.
Even worse, the exposure isn't limited to a single turbine on land. We've seen many cases where melting sections of a wind turbine that fell to the ground started fires on nearby brushland and triggered wildfires.
There is much more -much more- one could say on the subject, but I don’t want to spill all the beans on wind power, any more than you want to read about, so let’s leave it at this for now: Wind has spearheaded energy growth in the past few years. It goes without saying, though I’m happy to say it, with growth, size, and new frontiers, there are a number of risks that have proven that this market is one of the hardest to
Share, follow, stay tuned for more!